9) Accretion-ejection in GR-MHD (2017, 2018, 2019)
Here a fully relativistic approach is undertaken, applying resistive
GR-MHD simulations, launching outflows and jets from rotating black holes
and their surrounding accretion disk.
The simulations were done using our resistive version of the HARM code.
Publications are the following.
Vourellis, Fendt, Qian, Noble, ApJ 882, 2V (2019),
Qian, Fendt, Vourellis, ApJ 859, 28Q (2018),
Qian, Fendt, Noble, Bugli (2017).
Movie A: GR-MHD of accretion-ejection.
Color gradient shows mass density (left) and vertical speed v_z/c (right),
both in log scale.
Overlaid are poloidal magnetic field lines (black) and normalized poloidal velocity
vectors (i.e directions; pink).
Magnetic flux is advected towards the BH, angular momentum loss by the disk wind leads
to accretion. The poloidal magnetic field is bent torards the horizon (similar to
the classical Wald solution). Due to resistivity the anti-aligned magnetic field
along the equatorialplane inside the ISCO reconnects leading to a strongly
turbulent Blandford-Znajek outflow.
The disk outflow dominates the overall energy output.
Download
simulation run ( .avi format, Size = 80 MB)
Movie B: GR-MHD of accretion-ejection.
Same as above, but a sub-set of the innermost area.
The red circle is the horizon, the yellow ellipse the ergosphere, the dark
green circle indicates (!) the radius of the ISCO (located in the equatorial plane).
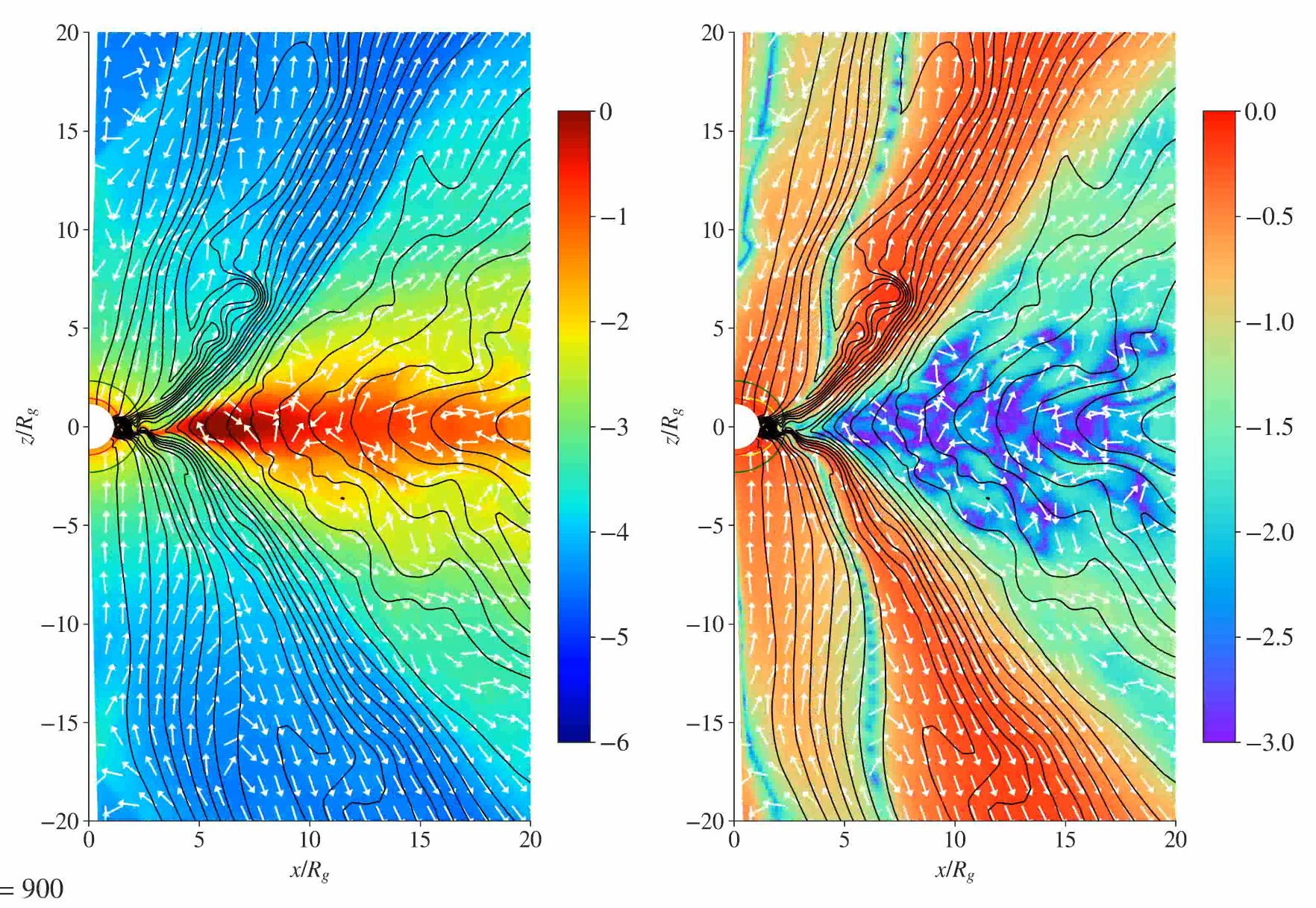
Download
simulation run ( .avi format, Size = 130 MB)
8) Large-scale MHD simulations of accretion-ejection incl. a disk dynamo (2014)
Now applying a spherical grid.
We also include a mean-field disk dynamo in the treatment and evolve
the jet-driving magnetic field from a toroidal seed field.
Here are movies of our MHD simulations perfomed with the PLUTO code.
Publications are the following.
Stepanovs, Fendt, Sheikhnezami, ApJ 796, 29S (2014)
for launching simulations with a disk dynamo-generated magnetic field, and
Stepanovs, Fendt, ApJ 825, 14S (2016),
Stepanovs, Fendt, ApJ 793, 31S (2014)
and
Sheikhnezami, Fendt, Porth, Vaidya, Ghanbari, ApJ 757, 65 (2012)
for launching simulations concentrating on the ejection process, and
Fendt, Sheikhnezami, ApJ 774, 12 (2011)
for truly bipolar simulations.
Movie A: Long-term evolution of the jet disk system.
Color gradient mass density.
Contours indicate three magnetic flux surfaces.
Initially magnetic flux diffuses outwards untill ejection has established
and removal of disk angular momentum leades to advection od mass and magnetic flux.
This simulations runs for more than 500.000 dynamical time steps on a
grid of more than 1000 inner disk radii.
(The image shows the density map (left) and the vertical velocity map (right).
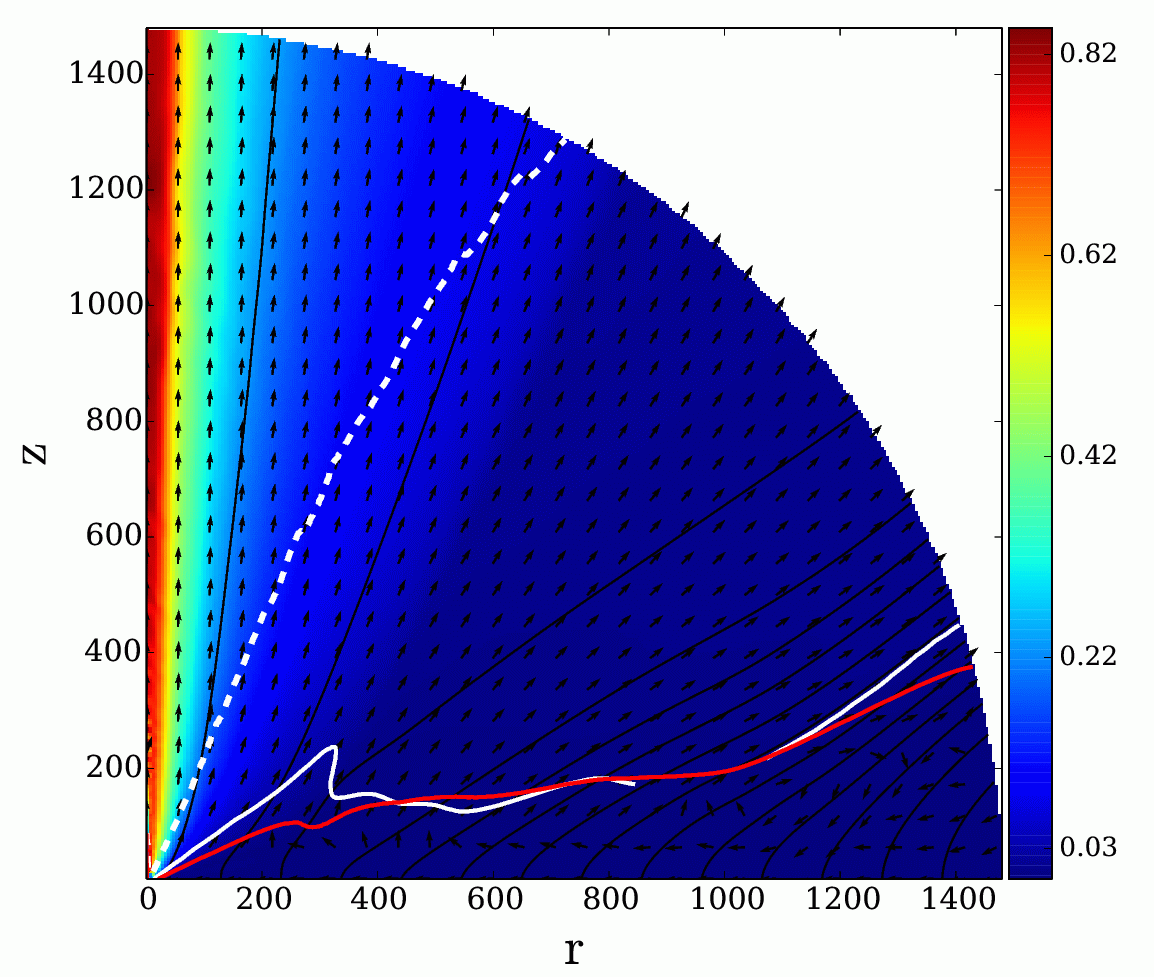
Download
simulation run ( .avi format, Size = 50 MB)
Movie B: A disk dynamo-generated jet magnetic field.
Color gradient mass density.
Contours indicate three magnetic flux surfaces.
These simulations start from a purely (weak) toroidal magnetic field.
The poloidal field driving the jet is generated by a alpha-Omega dynamo in the disk.
The dynamo effieciently generates a strong poloidal field in the inner
disk which then drives a jet. Poloidal magnetic flux loops are visible
in the outer disk.
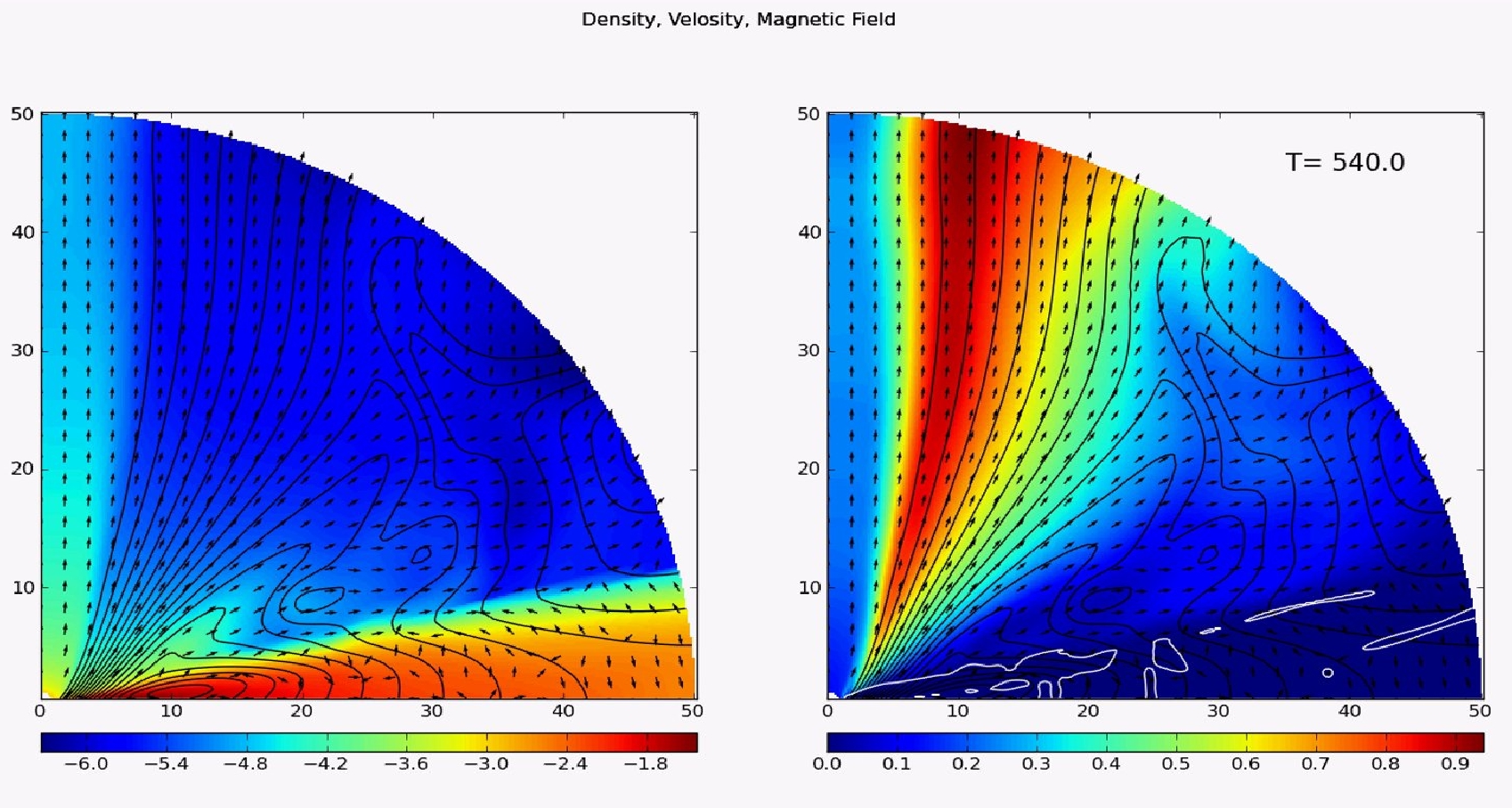
Download
simulation run ( .avi format, Size = 170 MB)
Movie C: Time-dependent knot ejection
Color gradient mass density.
Contours indicate three magnetic flux surfaces.
Time-dependent knot ejection can be triggered by time-dependent dynamo action.
Here we apply a dynamo toy model, by which the alpga-omega dynamo is switched
on and off within a period of 2000 dynamical time. Knots are ejcted from the
innermost disk in a similar time frame.
(pictures show the ejection of two knots within a short time frame of the
whole simulation run. Note the change of the color coding between the
snapshots and within the movie).
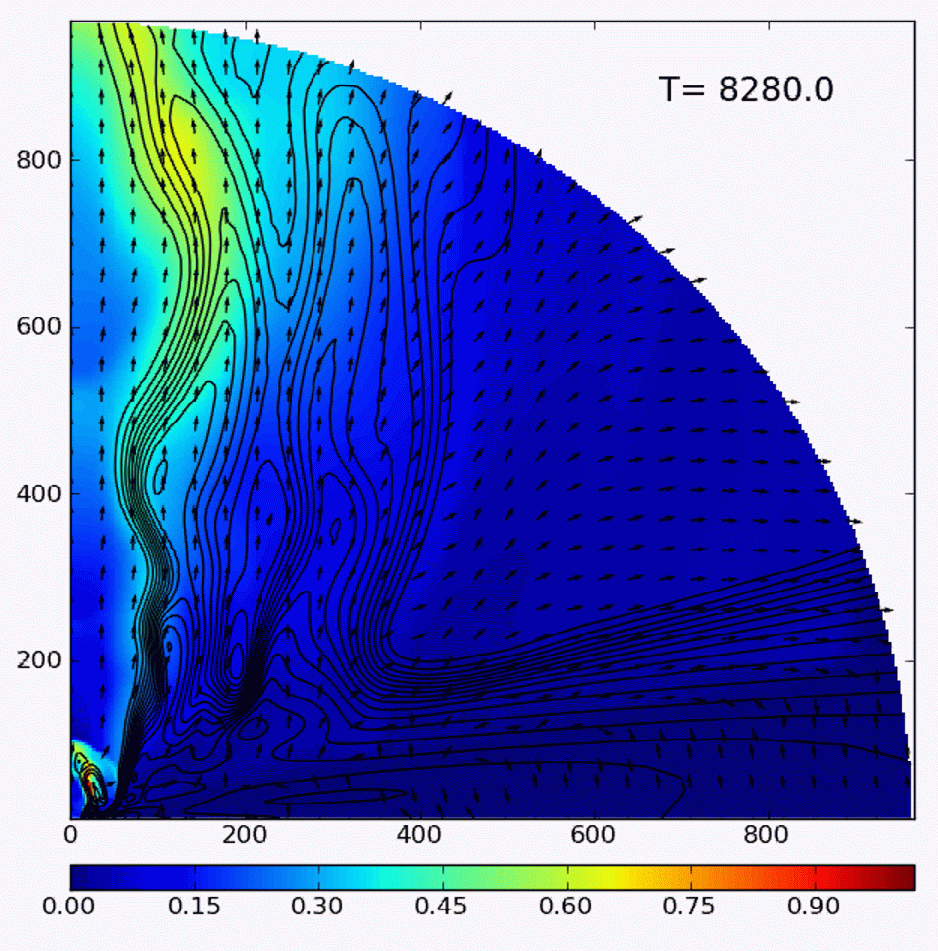
Download
simulation run ( .avi format, Size = 1 GB !!!!!)
7) MHD simulations of accretion-ejection structures (2012,2013)
Jets are launched by accretion disks. We numerical treat the
accretion-ejection system, including the evolution of the disk structure.
This finally provides the transfer rates for mass, energy and
angular momentum.
Here are movies of our MHD simulations perfomed with the PLUTO code.
See
Sheikhnezami, Fendt, Porth, Vaidya, Ghanbari, ApJ 757, 65 (2012)
for launching simulations concentrating on the ejection process, and
Fendt, Sheikhnezami, ApJ 774, 12 (2011)
for tryly bipolar simulations.
Movie A: Inner disk evolution - variation in the disk magnetization.
Color gradient mass density.
Contours indicate three magnetic flux surfaces.
In the picture below, coloured contours correspond to ONE magnetic flux
surface measured at different times.
Initially magnetic flux diffuses outwards untill ejection has established
and removal of disk angular momentum leades to advection od mass and magnetic flux.
A quasi steady state is reached after 2000 inner disk rotations (subject to
the contineous disk mass loss).
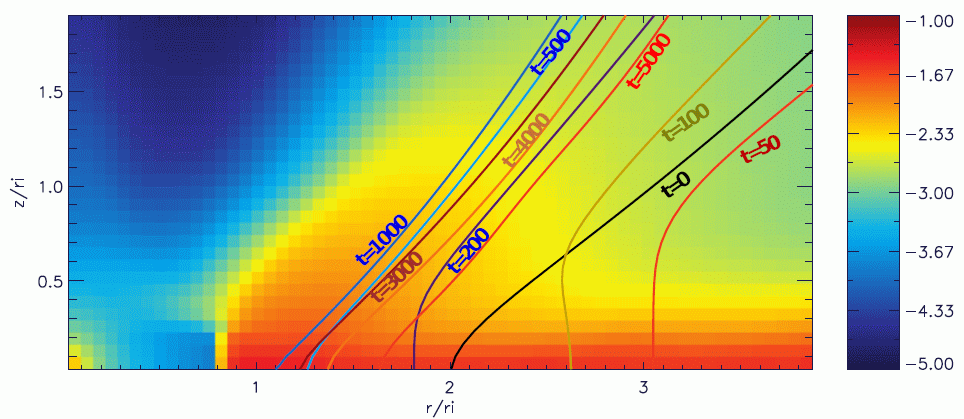
Download
simulation run ( .avi format, Size = 32 MB)
Movie B: Global evolution on one hemisphere.
Here we show the global evolution of the system -
launching from the disk into the jet, subsequent
acceleration and collimation into a jet.
Color gradient mass density.
Contours indicate magnetic flux surfaces.
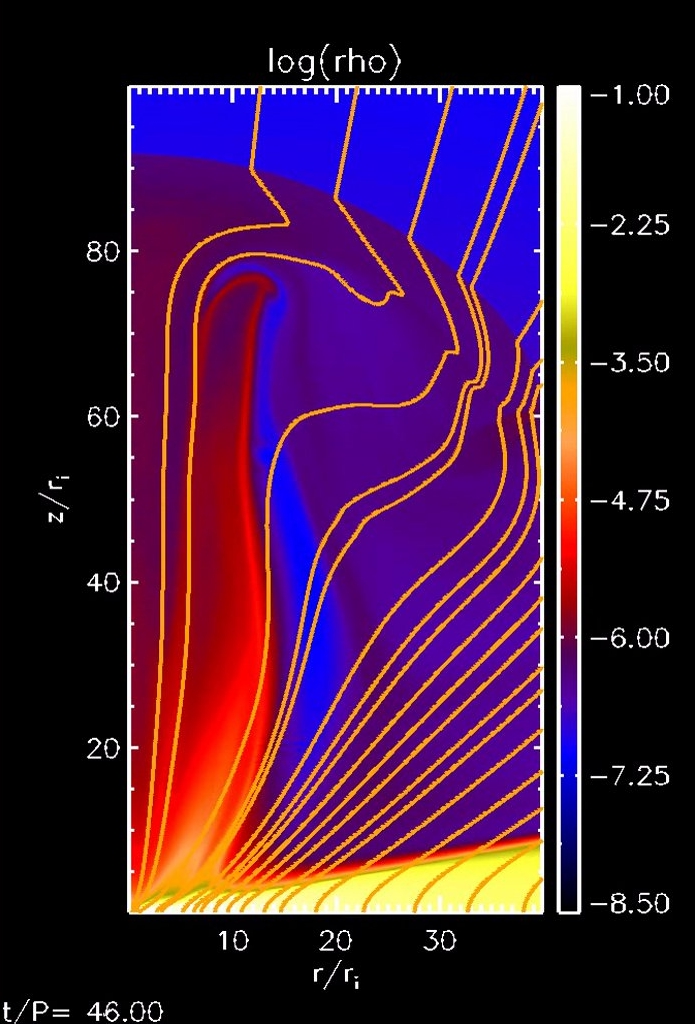
Download
simulation run ( .avi format, Size = 42 MB)
Movie C: Global evolution on both hemispheres.
This part of the project investigates the jet-counter jet
asymmetries by truly bypolar simulations.
The movie shows a symmetric evolution, th etest case of our
model setup.
Color gradient mass density.
Contours indicate magnetic flux surfaces.
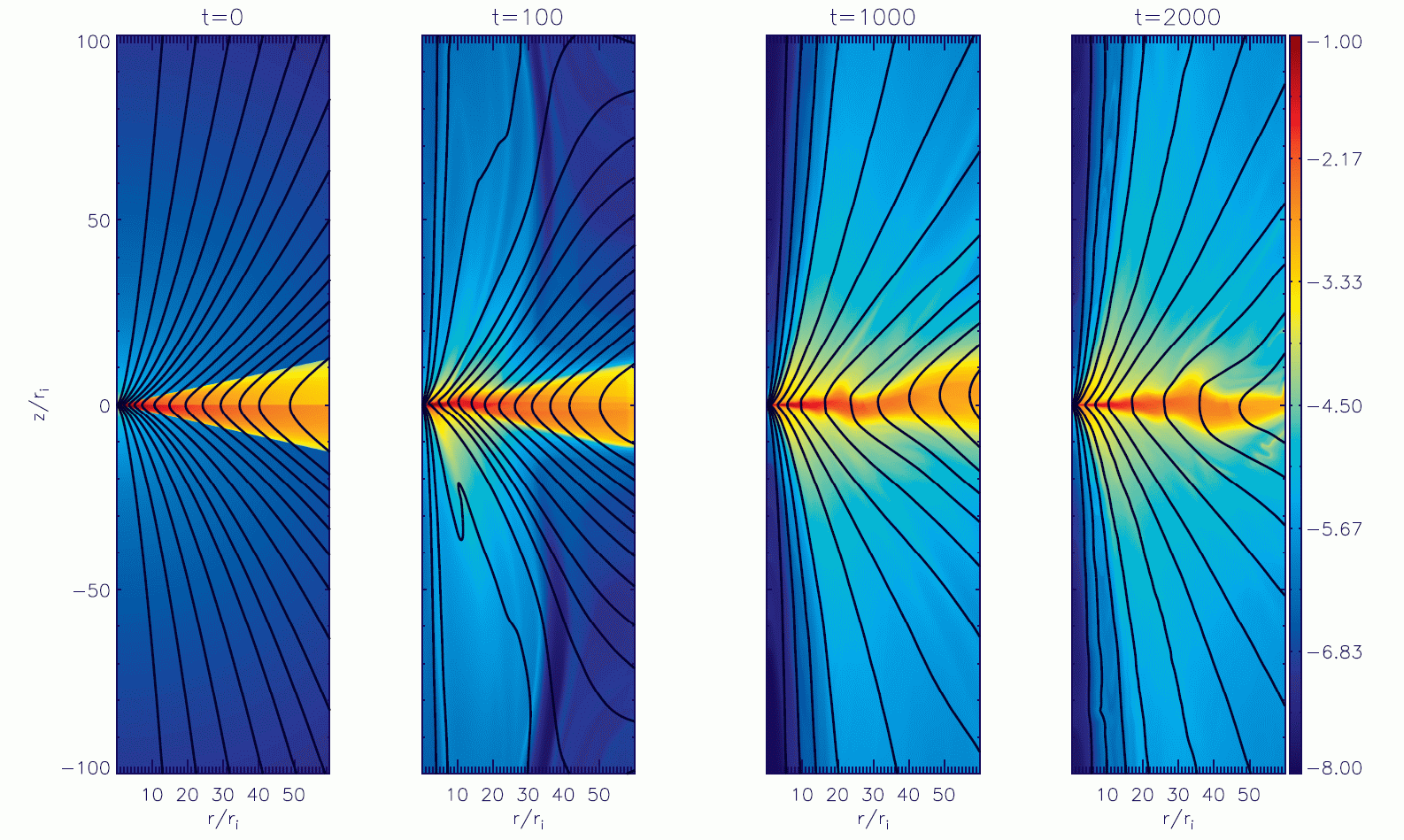
Download
simulation run ( .avi format, Size = 12 MB)
6) Relativistic MHD simulations of jets from compact sources (2010,2011)
Relativistic jets originate in the direct vicinity of the massive black holes residing in the
centers of most galaxies. Either they are launched as an initially sonic wind
of the accretion disk or they originate directly in the black holes ergosphere transforming
rotational energy into high Lorentz factors via magneto hydrodynamic processes.
Here are two movies of our RMHD simulations perfomed with the PLUTO code.
Our papers investigate time-dependent special relativistic MHD simulations
of jet formation from an accretion disk surface.
See
Porth, Fendt, ApJ 709, 1100 (2010)
for simulations of relativistic jet self-collimation, and
Porth, Fendt, Meliani, Vaidya, ApJ 737, 42 (2011)
for mock observations of the simulated MHD data.
Movie A: Relativistic disk wind in a hourglass magnetosphere.
Color gradient indicates the vertical velocity in units of c.
Contours are: Field lines (white); electric current lines (green); light
cylinder (blue).
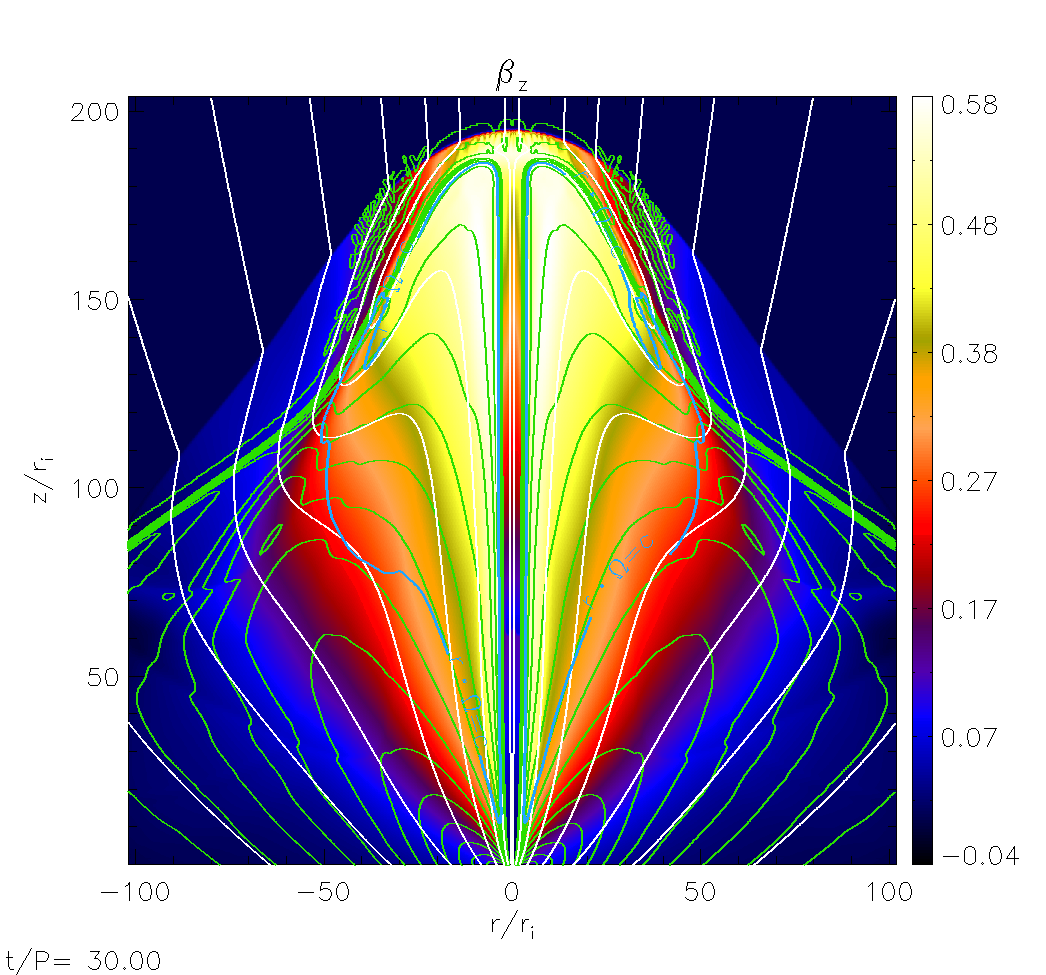
Download
simulation run ( .avi format, Size = 6.7 Mb)
Movie B: As the previous one but for a highly inclined split monopole geometry.
The field-lines are pushed back into the disk surface and hence no steady state
is established.
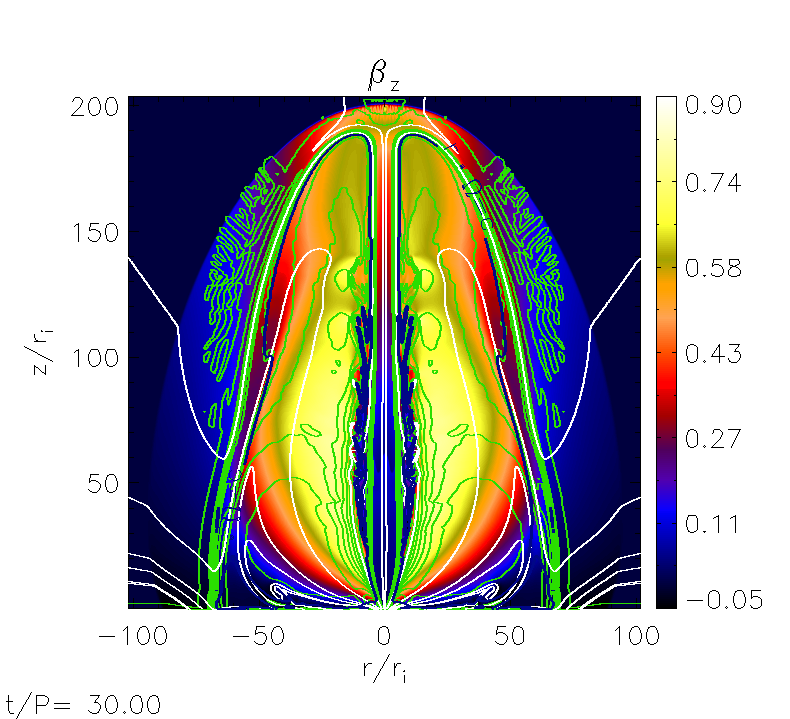
Download
simulation run ( .avi format, Size = 6.7 Mb)
5) MHD simulations: jets from massive YSO affected by radiation pressure (2011)
This paper investigates time-dependent evolution of a superposed
disk magnetic field with a stellar dipolar magnetosphere.
See
Vaidya, Fendt, Beuther, Porth, ApJ 742, 56 (2011).
Movie A: MHD jet under stellar radiation pressure
Colors indicate velocity with a scale factor of 163 km/s.
Black Lines are magnetic field lines.
Radiation line-forces switched on after 319 disk rotations
Parameters: Mstar = 30 Msun, Rin = 1 AU, Alpha = 0.55, Qo = 1400.0, rho_0 = 5.0e-14 g/ccm
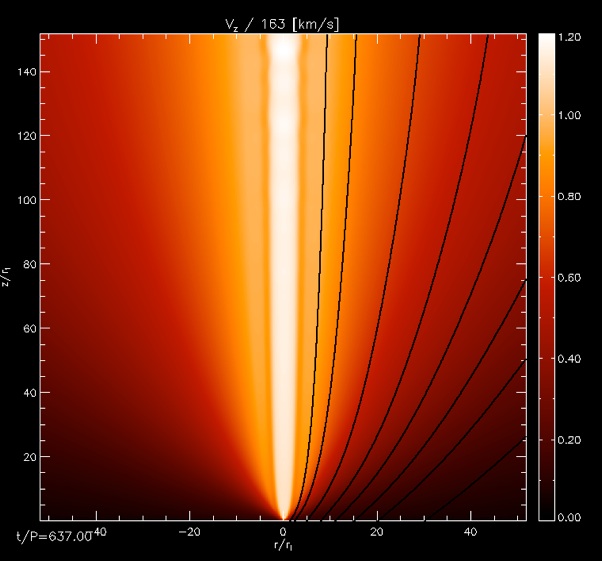
Download
simulation run ( .avi format, Size = 6.7 Mb)
Movie B: MHD jet under radiative force of disk